Quantum computing is rapidly transforming the landscape of technology and science, with qubits at the core of this revolution. Unlike classical bits, qubits leverage the principles of quantum mechanics, such as superposition and entanglement, to perform complex computations at unprecedented speeds. As research and development in qubit technology advance, key innovations are overcoming challenges in stability and error correction, paving the way for more powerful and efficient quantum computers. This article explores the fundamental role of qubits in quantum computing, the differences between qubits and classical bits, and the cutting-edge technologies driving their implementation, as well as their current applications and future prospects.
gameslino.com will take you through an extensive exploration of this topic.
1. Definition and Role of Qubits in Quantum Computing
Qubits, or quantum bits, are the fundamental units of information in quantum computing, analogous to classical bits in traditional computing. However, unlike classical bits, which can exist only in a state of 0 or 1, qubits can exist in multiple states simultaneously thanks to the principle of superposition. This ability allows quantum computers to process vast amounts of data simultaneously, exponentially increasing computational power.
The role of qubits in quantum computing extends beyond just holding information. Through quantum entanglement, qubits can become interconnected, meaning the state of one qubit is directly related to the state of another, even when separated by large distances. This interconnectivity allows for faster information transfer and more complex computations. In essence, qubits are the building blocks that enable quantum computers to solve problems that are currently intractable for classical computers, such as factoring large numbers, simulating complex molecules, and optimizing large systems.
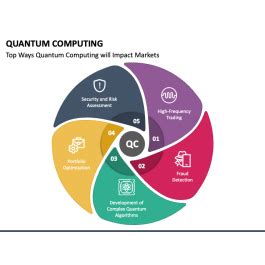
2. Differences Between Qubits and Classical Bits
Classical bits are the basic units of information in traditional computing, representing data as either 0 or 1. These binary states are fixed and mutually exclusive, meaning a classical bit can only be in one state at any given time. This binary system underpins all classical computing processes, limiting the speed and complexity of calculations.
In contrast, qubits operate on the principles of quantum mechanics, allowing them to exist in a state of 0, 1, or any quantum superposition of these states. This means that a qubit can process multiple possibilities simultaneously, vastly increasing computational power and efficiency. Additionally, qubits can be entangled, creating a strong correlation between their states even when separated by distance, something impossible with classical bits.
The primary difference lies in this quantum capability, enabling qubits to perform parallel computations, whereas classical bits can only handle one computation at a time. This fundamental difference is what gives quantum computing its potential to outperform classical systems in certain tasks.
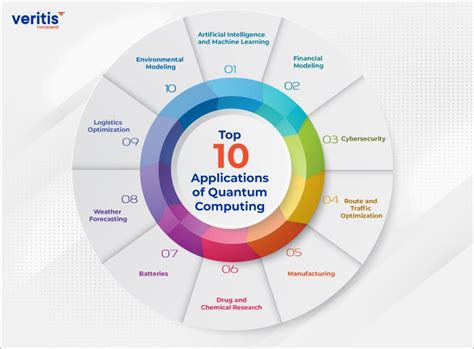
3. Quantum Entanglement and Qubit Superposition
Quantum entanglement and superposition are two foundational principles that give qubits their extraordinary capabilities in quantum computing. Superposition allows a qubit to exist in a combination of states—0 and 1—simultaneously. This is unlike classical bits, which are restricted to being either 0 or 1 at any given time. Because qubits can process multiple states at once, they enable quantum computers to handle vast amounts of data and perform complex calculations much faster than classical computers.
Quantum entanglement is another key concept where two or more qubits become interconnected such that the state of one instantly influences the state of the other, no matter the distance between them. This phenomenon creates a powerful link between qubits, enabling them to work together in ways that classical bits cannot.
When combined, superposition and entanglement allow quantum computers to perform multiple calculations simultaneously and efficiently solve problems that are currently impossible for classical computers. These properties make qubits essential for advancing quantum algorithms, cryptography, and other cutting-edge applications in technology and science.
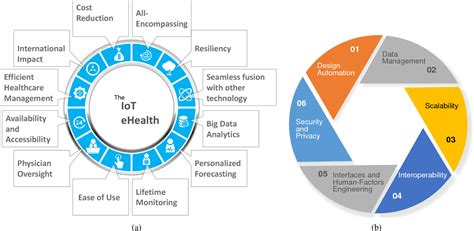
4. Key Technologies for Qubit Implementation (e.g., superconducting qubits, trapped ions)
The implementation of qubits in quantum computing relies on several advanced technologies, each with its unique advantages and challenges. Among the most prominent are superconducting qubits and trapped ions, both of which represent different approaches to harnessing quantum mechanics for computation.
Superconducting qubits are created using tiny superconducting circuits that can maintain quantum states with minimal energy loss. These qubits operate at extremely low temperatures, close to absolute zero, to exploit superconductivity—a state where electrical resistance drops to zero. This technology is favored by many leading companies, such as IBM and Google, due to its scalability and the ability to integrate with existing semiconductor fabrication techniques. However, maintaining the necessary low temperatures and minimizing quantum decoherence—loss of quantum information—remain significant challenges.
Trapped ion qubits, on the other hand, use individual ions confined in electromagnetic fields. These ions are manipulated using laser pulses to perform quantum operations. Trapped ion technology offers high levels of qubit coherence and precision, making it ideal for highly accurate quantum computations. However, scaling up this technology to handle large numbers of qubits is complex and requires sophisticated equipment.
Other emerging technologies include topological qubits, which promise to be more stable and less prone to errors, and photonic qubits, which use light particles to transmit quantum information. Each technology represents a different pathway to building more powerful and reliable quantum computers.
5. Challenges in Qubit Stability and Error Correction
Qubit stability and error correction are among the most significant challenges in the development of quantum computing. Qubits are highly sensitive to environmental disturbances such as temperature fluctuations, electromagnetic noise, and even cosmic rays. These disturbances can cause qubits to lose their quantum state, a phenomenon known as decoherence, which leads to errors in computation.
One of the primary challenges is maintaining qubit coherence over a sufficient period to perform meaningful computations. The fragile nature of qubits makes them prone to errors, necessitating sophisticated error correction methods. Quantum error correction is more complex than its classical counterpart because it must preserve the delicate quantum states without directly measuring or disturbing them.
Techniques such as surface codes, which involve using multiple physical qubits to represent a single logical qubit, are being developed to manage and correct errors. However, implementing these techniques requires a significant increase in the number of qubits, which adds to the complexity and resource demands of quantum computers. Despite these challenges, ongoing research continues to improve qubit stability and develop more effective error correction methods, moving closer to practical quantum computing.
6. Advances in Qubit Research and Development
Recent years have seen significant advances in qubit research and development, driving the progress of quantum computing closer to practical applications. Researchers are exploring various materials and techniques to improve qubit coherence times, reduce error rates, and increase the scalability of quantum systems.
One notable area of progress is in superconducting qubits, where companies like IBM and Google have made strides in creating more stable and reliable qubit designs. These advances have led to the demonstration of quantum supremacy, where quantum computers outperform classical counterparts in specific tasks. Additionally, advancements in quantum error correction methods, such as the development of more efficient surface codes, are enhancing the reliability of quantum computations.
In the field of trapped ion qubits, researchers are refining ion trapping and manipulation techniques, achieving higher levels of precision and control. This has enabled more complex quantum algorithms to be executed with greater accuracy.
Emerging technologies like topological qubits, which aim to be inherently more resistant to errors, and photonic qubits, which use light for quantum information processing, are also gaining traction. These innovations, combined with improvements in qubit connectivity and control, are paving the way for more powerful and scalable quantum computers, opening new possibilities in fields ranging from cryptography to material science.
7. Major Companies and Their Qubit Technologies
Several major companies are at the forefront of developing qubit technologies, each employing different approaches to advance quantum computing. IBM is a leading player, focusing on superconducting qubits. Their quantum computing platform, IBM Quantum, utilizes these qubits to explore and solve complex problems, and IBM has already demonstrated significant milestones in quantum supremacy.
Google, another key player, is renowned for its use of superconducting qubits. The company’s Sycamore processor achieved a landmark moment in quantum computing by solving a specific problem faster than the most advanced classical supercomputers. Google’s ongoing research aims to enhance qubit stability and expand quantum computing capabilities.
In the realm of trapped ions, IonQ is a prominent company. They leverage laser manipulation of individual ions to perform quantum computations, offering high precision and long coherence times. IonQ’s technology is known for its potential in achieving scalable and highly accurate quantum systems.
Other notable companies include Rigetti Computing, which also utilizes superconducting qubits, and D-Wave, which focuses on quantum annealing techniques. Each company’s approach reflects diverse strategies to address the challenges of qubit technology and drive the future of quantum computing.
8. Impact of Qubits on Quantum Algorithms and Computing Power
Qubits have a profound impact on quantum algorithms and computing power, fundamentally changing how complex problems are approached and solved. Unlike classical bits, which handle information linearly, qubits leverage superposition and entanglement to process multiple possibilities simultaneously. This capability allows quantum algorithms to perform parallel computations, significantly enhancing computational efficiency.
One of the key impacts of qubits is seen in quantum algorithms such as Shor’s algorithm, which can factor large numbers exponentially faster than the best classical algorithms. This has profound implications for cryptography, potentially revolutionizing data security. Another notable algorithm is Grover’s algorithm, which offers quadratic speedup for unstructured search problems, making it valuable for various applications, from database searches to optimization problems.
The power of qubits also enables quantum computers to tackle problems that are currently intractable for classical systems. For instance, simulating complex quantum systems in chemistry and materials science becomes feasible, allowing for breakthroughs in drug discovery and new material development. Additionally, quantum machine learning algorithms can process and analyze vast datasets more efficiently, opening new frontiers in AI and data science.
Overall, the unique properties of qubits expand the computational capabilities of quantum computers, offering exponential speedups and new possibilities across various fields of science and technology.
9. Current Applications of Qubits in Quantum Computing
Currently, qubits are being utilized in several cutting-edge applications of quantum computing, showcasing their potential to revolutionize various fields. One prominent application is in quantum simulation, where qubits are used to model complex quantum systems that are difficult to simulate with classical computers. This capability is particularly valuable in chemistry and material science, enabling researchers to explore molecular structures and reactions with unprecedented accuracy, which can accelerate drug discovery and the development of new materials.
Another significant application is in optimization problems. Quantum algorithms leverage qubits to solve complex optimization challenges more efficiently than classical methods. This includes applications in logistics, finance, and supply chain management, where quantum computing can optimize routes, portfolios, and resource allocation.
Quantum cryptography is also benefiting from qubit technology. Qubits enable the development of quantum key distribution (QKD) systems, which promise enhanced security by detecting eavesdropping attempts and ensuring secure communication channels.
Moreover, qubits are being explored in quantum machine learning, where they enhance the processing of large datasets and improve the efficiency of AI algorithms. This could lead to advancements in data analysis, pattern recognition, and predictive modeling. As quantum computing technology evolves, these applications are expected to expand, offering transformative solutions across various industries.
10. Future Prospects and Innovations in Qubit Technology
The future of qubit technology holds exciting prospects and innovations poised to further revolutionize quantum computing. Researchers are actively exploring new materials and techniques to enhance qubit performance, aiming to increase coherence times and reduce error rates. One promising direction is the development of topological qubits, which are designed to be inherently more stable and resistant to errors due to their unique quantum properties.
Advancements in qubit connectivity and scalability are also on the horizon. Improved methods for integrating and linking qubits could lead to larger, more powerful quantum computers capable of solving even more complex problems. Innovations in quantum error correction techniques are expected to further enhance the reliability of quantum computations, making practical quantum computing more feasible.
Additionally, hybrid quantum-classical computing models are being developed to leverage the strengths of both systems, potentially leading to more efficient problem-solving approaches. As research continues and technology evolves, these innovations will pave the way for groundbreaking applications across various scientific and industrial fields, transforming how we approach complex computational challenges.
Qubits are the cornerstone of quantum computing, offering unparalleled computational power through superposition and entanglement. Despite challenges in stability and error correction, advancements in qubit technologies and research are driving significant progress. As innovations continue to unfold, qubits will play a crucial role in solving complex problems, revolutionizing industries, and unlocking new possibilities in science and technology.
gameslino.com